Putting the Carbon Genie to Work
January 20, 2021
Our climate crisis is an energy crisis where use of coal, oil, and natural gas has led to incredible progress as well as record-breaking levels of CO2 in the atmosphere. But fossil fuels aren’t “bad,” per se. Especially in Louisiana, where the petrochemical industry provides jobs and creates essential products we rely on every day. LSU researchers in multiple colleges are now working on new solutions together with industry and government to lower CO2-concentrations in the atmosphere. This can be done by capturing CO2 (right at the emissions source, or directly from the air), storing it safely underground; and working with natural systems—our farms and wetlands—to stockpile excess carbon. We can also use CO2 as a feedstock in chemical manufacturing as a sustainable substitute for oil and natural gas, and as a tool to get more oil from “depleted” or abandoned reservoirs.
Jump to Carbon Capture, Use and Storage Jump to Enhanced Oil Recovery Jump to Blue Carbon
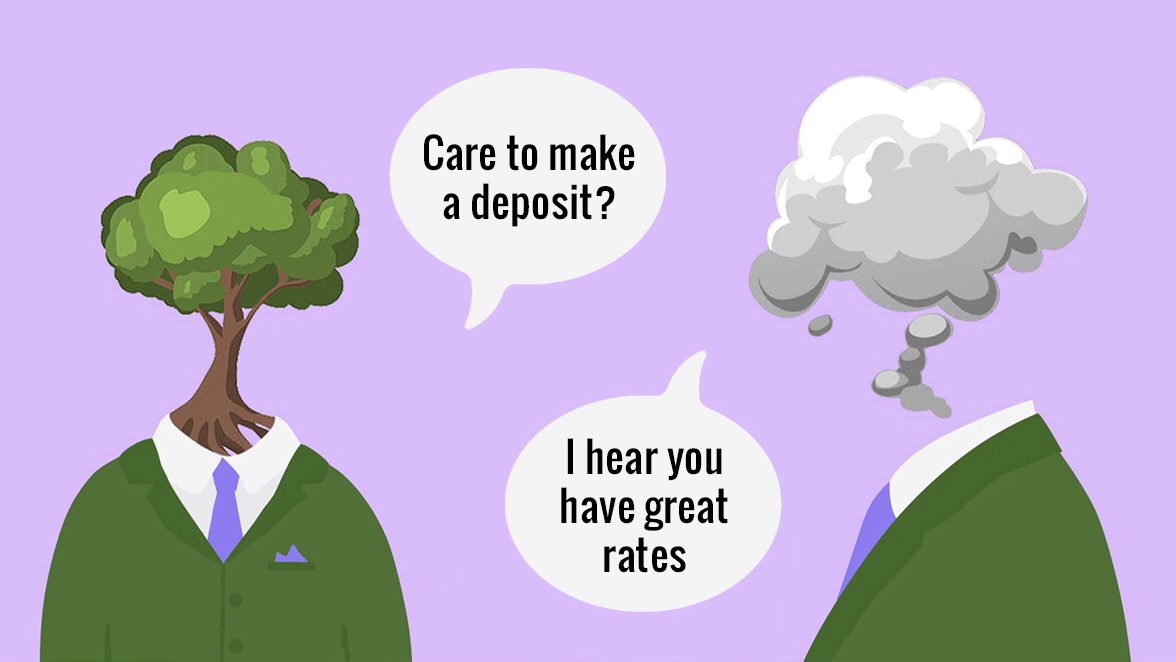
To lower carbon dioxide levels in the atmosphere, we can work with natural systems, such as wetlands and mangroves, which can store large amounts of carbon and keep it isolated from the ever-churning carbon cycle. But there is also a lot more we can do. We can decrease our reliance on fossil fuels by improving and implementing renewable energy alternatives at scale, while continuing to use fossil fuels in more efficient and targeted ways that can help—not hurt—the environment.
– Elsa Hahne
Recognized as the main driver behind global warming and climate change, the concentration of carbon dioxide (CO2) in the atmosphere sets new records each year. The effects are clear in Louisiana and in coastal communities around the world, where higher temperatures and sea level rise lead to stronger storms, more frequent flooding, and other costly and sometimes life-threatening problems.
LSU researchers in several colleges are now merging science, technology, engineering, law, and environmental and coastal sciences to help find solutions to what Assistant Professor Brian Snyder in the LSU College of the Coast & Environment calls “the defining problem of the 21st Century”—curbing CO2 emissions while also actively removing CO2 from the atmosphere through new, innovative technologies and by setting a price on carbon, the most basic building block for all life on Earth—at the center of today’s energy politics.
Nine LSU researchers have joined committees and advisory groups for Louisiana Governor John Bel Edwards’s new Climate Initiatives Task Force, announced last November, several of whom directly work on limiting CO2 emissions: Mehdi Zeidouni and Richard Hughes (petroleum engineering), Kalliat Valsaraj (chemical engineering), Chang Jeong, Richard Keim, and Naveen Adusumilli (agriculture), Craig Colten (geography and anthropology), Nick Bryner (law), and Jim Richardson (business).
Today in the U.S., 80% of the energy we consume comes from fossil fuels—renewable energy accounts for just over 10%.
The LSU Center for Energy Studies also recently signed a contract with the Governor to help estimate Louisiana’s official greenhouse gas inventory by June 2021. This will be a key tool for the Climate Initiatives Task Force in assessing the effectiveness of various policy proposals to reduce or mitigate emissions in the state.
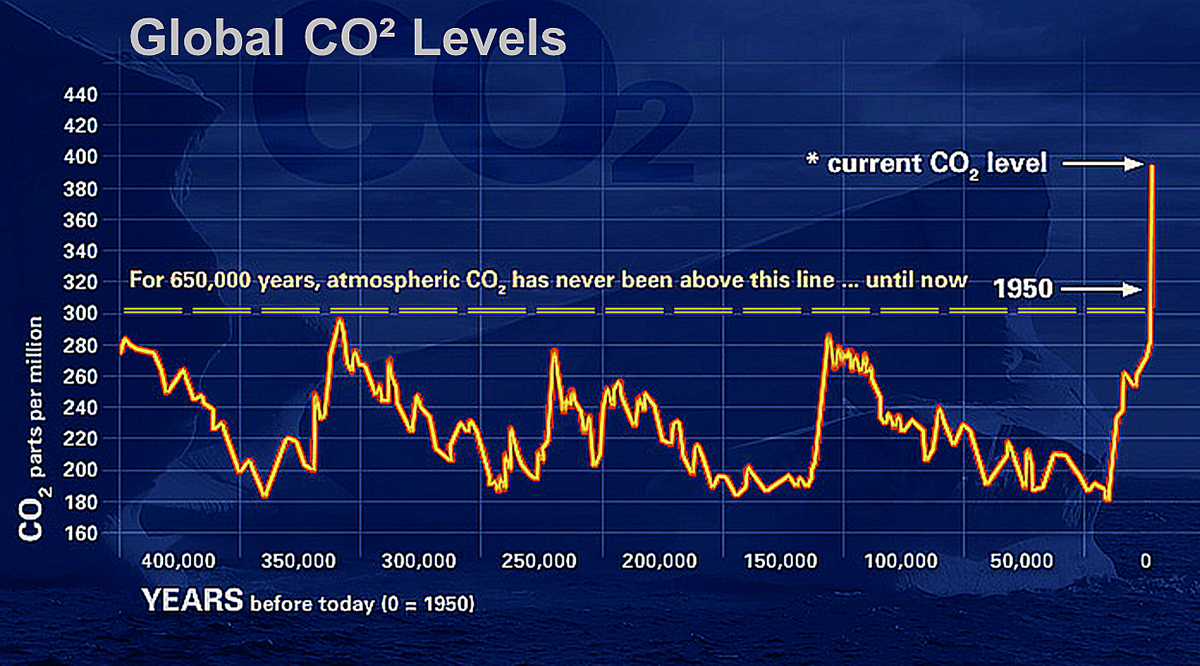
Global CO2-levels have reached a historic high since there have been people on the planet.
– NASA
Surprisingly, it wasn’t until the Obama administration that CO2 was considered a pollutant under the Clean Air Act. Part of the reason it took so long—after all, we’d been tracking greenhouse gases for over 50 years—is that CO2 is a natural part of life. We exhale it, plants use it to grow; we wouldn’t have food without it. The problem is that we now have too much CO2 in the atmosphere due to our still-rising consumption of fossil fuels (petroleum, natural gas, coal), which has increased eight-fold around the globe since 1950.
Today in the U.S., 80% of the energy we consume comes from fossil fuels—renewable energy accounts for just over 10%. For power generation, the numbers shift a little. Close to 20% of all electricity comes from renewable sources, while natural gas alone accounts for almost 40% in the nation (and more than 70% in Louisiana, at the epicenter of U.S. natural gas production). Natural gas is expected to hold at this number until 2050, by which time the Paris Agreement demands net-zero greenhouse gas emissions, lest we cannot keep the average global temperature increase under 1.5 degrees Celsius, considered a critical threshold.
The key to decreased use of fossil fuels lies in conservation, through smarter, more targeted use and higher energy efficiency, as well as better and more widespread adoption of renewables. This is why LSU researchers are innovating in energy-saving technologies, carbon capture and reuse, long-term solutions for storing CO2 safely underground and in natural ecosystems, as well as improved solar, wind, biofuel, and fuel cell technologies to help meet growing energy demands. Who doesn’t want air conditioning in the summer and, as summers get hotter, maybe a little more air conditioning?
Since we will continue to rely on fossil fuels for some time, several LSU researchers are working on ways to use them in safer and more efficient ways.
A major challenge over the next few decades, as we continue to rely on fossil fuels while ramping up green energy capacity, is that higher standards of living are intrinsically linked to higher energy consumption. While we’d ideally want everyone in Louisiana and a world population that has tripled in size since 1950 to “do better” than previous generations, this ups the ante as far as how much renewable energy can be made available—to everyone, and fast.
Since we will continue to rely on fossil fuels for some time, several LSU researchers are working on ways to use them in safer and more efficient ways. This is where carbon capture comes in.
Carbon Capture, Use, and Storage
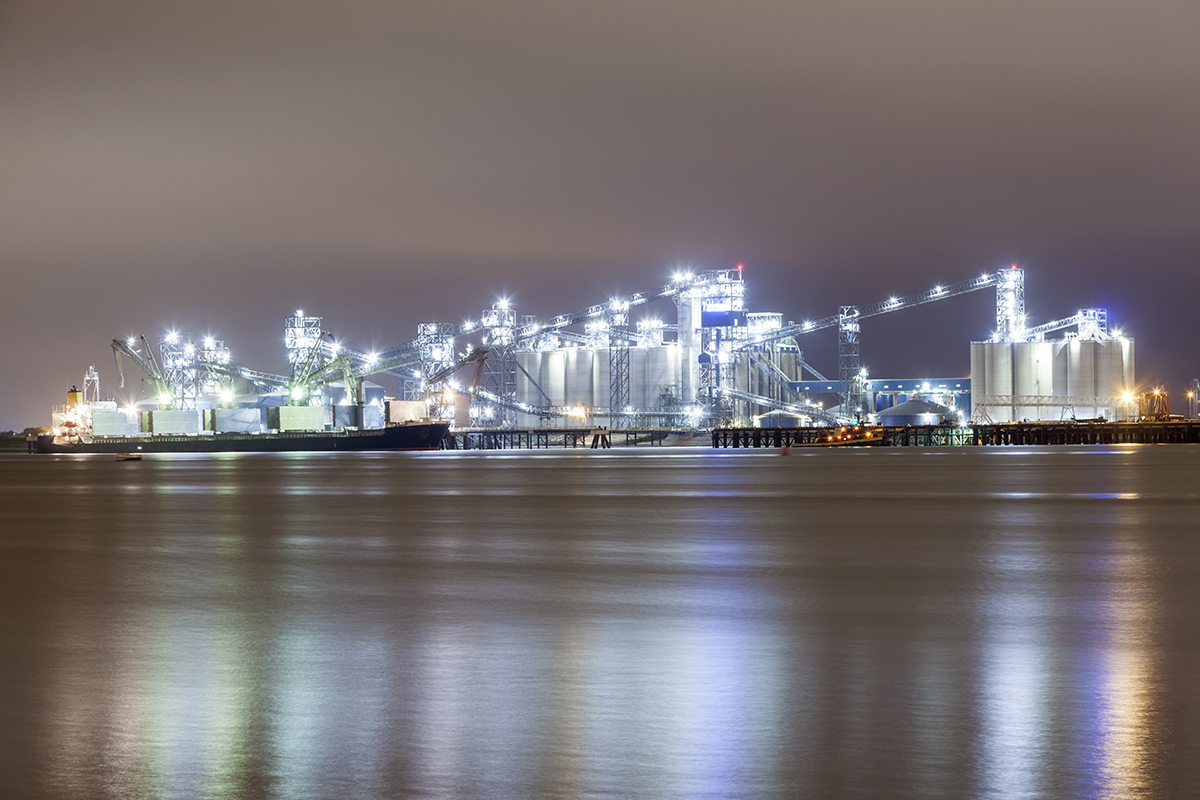
The petrochemical industry in Louisiana is geographically concentrated, which can allow for efficent and cost-effective carbon dioxide capture before it goes into the atmosphere as part of industrial emissions.
Carbon capture is a portfolio of emerging technologies that do either of two things—prevent CO2 emissions from going into the atmosphere or remove some of the CO2 that was already emitted. At present, carbon capture is the only tool available to us to potentially reverse global warming and achieve not just net-zero carbon emissions, which Governor John Bel Edwards has set as a goal for Louisiana by 2050, but possibly net-negative carbon emissions.
While Louisiana currently ranks fourth among U.S. states for carbon-intensity of the economy and per-capita CO2 emissions, the state could potentially “flip the switch” and instead become a leader for the nation in carbon capture, said John Flake, Jay Affolter Endowed Professor and chair of the LSU Cain Department of Chemical Engineering.
“Because of our existing resources, I think Louisiana is the perfect place for this,” he said. “The infrastructure is already here, the expertise is already here, the low-cost energy is here, and the carbon dioxide is here.”
LSU environmental sciences professor Brian Snyder agrees. He, Brittany Tarufelli, and David Dismukes in the LSU Center for Energy Studies have run a cost analysis for almost 600 industrial facilities, including dozens in Louisiana, to see how profitable it would be for them to retrofit their buildings for successful carbon capture right at the source. As the U.S. Congress expanded the 45Q tax credit program three years ago to encourage more carbon capture, adoption of these new technologies became a winning proposition for many—especially petrochemical facilities in Louisiana.
As the U.S. Congress expanded the 45Q tax credit program three years ago to encourage more carbon capture, adoption of these new technologies became a winning proposition for many—especially petrochemical facilities in Louisiana.
“The industrial sources are the ones that have high-purity, concentrated CO2,” Snyder explained. “For them, carbon capture can be profitable, but they need help connecting the dots between capture, transportation, use, and storage, and this is why we’ve hosted workshops in the last couple of years for industry and state and local government to do exactly that.”
Snyder has also researched how to best capture carbon dioxide directly from the air, using light, electricity, methane, and sulphur as alternative ways to help drive the process. The greatest challenge of capturing CO2 straight from the atmosphere is that it goes against the laws of thermodynamics to force concentration of CO2, rather than the opposite. Therefore, it takes a lot of energy—and unless that energy comes from renewable sources and instead comes from burning fossil fuels, “it defeats the whole purpose,” Snyder said. Well, unless the CO2 is captured at the source, at least.
Snyder calculated that it would cost more than half of all energy that was consumed in 2018 to direct-air-capture the CO2 emitted that year. So, best to do it where it’s cheaper and easier, such as in the Louisiana chemical corridor, along the Mississippi River between Baton Rouge and New Orleans, and around Lake Charles. Because the biggest share of CO2 emissions in Louisiana comes from industrial sources (not transportation and power generation, as in other states), carbon capture is more affordable here—the U.S. Environmental Protection Agency, or EPA, estimated a comparative cost of $20-35 per metric ton of CO2 emitted in Louisiana versus $60-160 elsewhere.
Where will carbon to make ethylene to make essential products come from, once we run out of fossil fuels?
Emissions from chemical manufacturing—a colossal, growing industry that employs 15-20% more Louisianans than it did just 10 years ago—are expected to keep rising in coming years as more people rely on more products created from key chemicals, such as ethylene, the simplest alkene, which has a double bond between two carbon atoms each connected to hydrogen, as C2H4. The production of ethylene exceeds that of any other organic compound in the world and Flake estimates it to account for almost half of all chemical manufacturing in Louisiana. At present, it’s made from oil or natural gas in petrochemical plants through something called a cracking process.
“Ethylene is like your most basic Lego block,” Flake said. “Once you make ethylene, you can create almost anything. That double bond makes it very reactive. You can make it grab a hydroxide, or a polymer—anything you want.”
Ethylene is used to make a host of products—plastic milk jugs, polyester fabrics, phones, antifreeze, PVC pipes, intubation devices, tennis shoes, insulation, alcohols, and liquid detergents, such as shampoo and soap.
“If you wash your clothes with detergent in Washington, that probably started as natural gas somewhere in Louisiana,” Flake said. “It was probably converted to ethylene in Lake Charles and then to a long-chain alcohol there, maybe next door, and then it probably went to Procter & Gamble in Pineville, across the Red River from Alexandria.”
“Plastics have a negative connotation, and for good reason,” Flake continued. “But while I think most of us would be happy to go without plastic bags at the grocery store, I’m not so willing to go without clean clothes. Also, we need plastics for certain things, like in hospitals—and cars! I mean, what are you going to do? Make a dashboard out of wood or glass? That’s just laughable.”
Dismukes drives this point home as well. Renewable energy is great for power generation, but you need carbon to make some of the products we rely on every day, and, especially when it comes to plastics, could recycle more efficiently:
“You can’t make plastics with a windmill.”
Flake’s solution to lowering CO2-emissions from petrochemical facilities that make ethylene is to use carbon capture, on the one hand, and also use the CO2 itself as a feedstock, or raw material, to make ethylene.
Flake’s solution to lowering CO2-emissions from petrochemical facilities that make ethylene is to use carbon capture, on the one hand, and also use the CO2 itself as a feedstock, or raw material, to make ethylene. And instead of driving that conversion with heat (from natural gas), he’s finding better ways to do it using electrical energy from renewable sources by developing new electric catalysts.
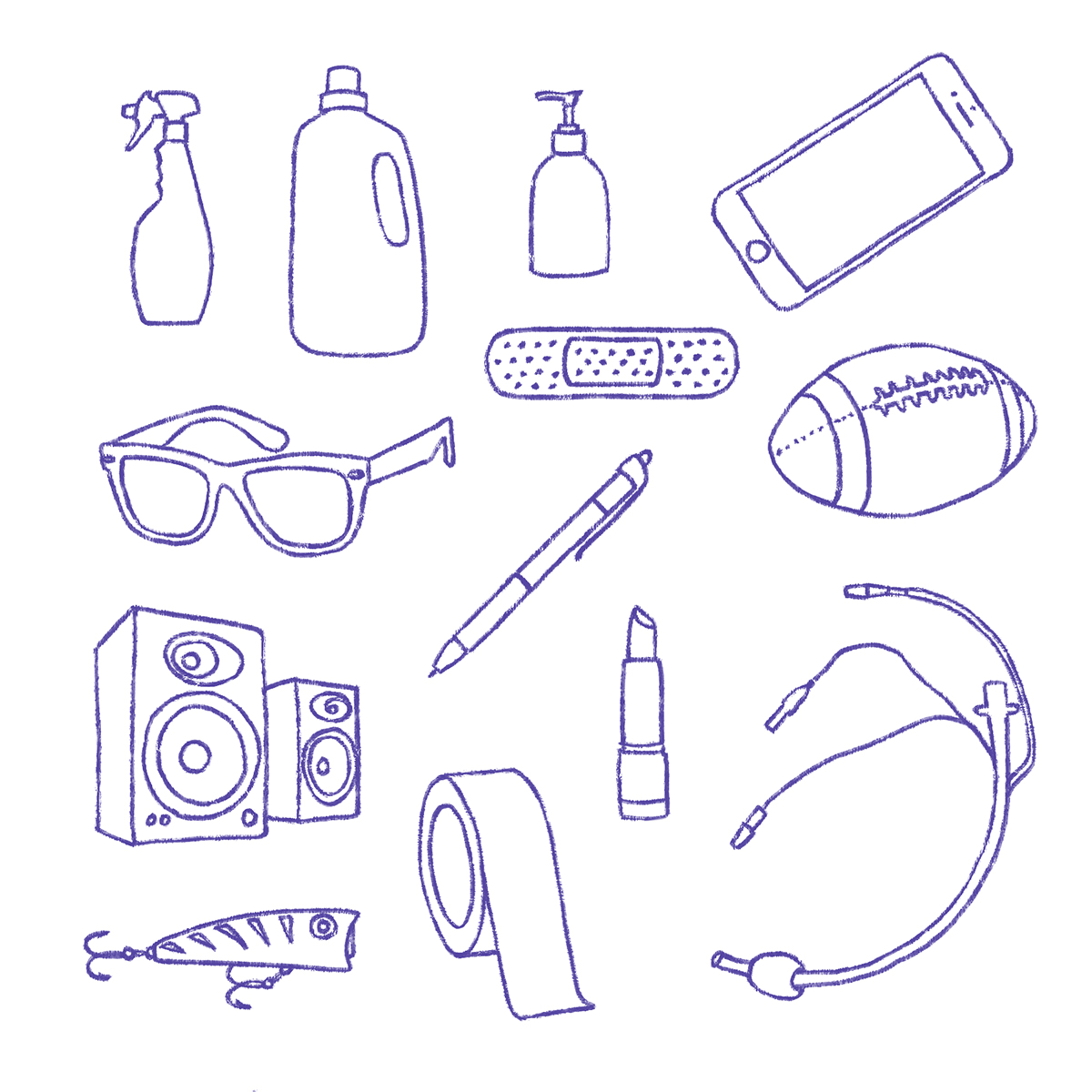
Many of the products we use every day are made from ethylene, which in turn is made from petrochemicals—oil and natural gas. By using carbon dioxide as a feedstock instead of fossil fuels to make ethylene, LSU chemical engineers John Flake is working to make Louisiana’s growing chemical manufacturing industry more sustainable.
– Elsa Hahne
“Outside of a few reactions, we haven’t really figured out how to leverage electrical energy to drive chemical reactions in the same way we’ve figured out how to use temperature, so that’s what I’m working on,” Flake said. “And if you’re going to build a new ethylene plant that gets its carbon from carbon dioxide and energy from renewables, Louisiana is a great place to do it.”
Another consideration is where carbon is going to come from once we run out of fossil fuels, which scientists agree will happen “at some point soon.”
“Well, from CO2. We have a lot of it.”
Scientists at LSU are also working on this problem by developing new catalysts that use electricity, water, and CO2 to make fuels or other carbon-containing products. These building blocks can be used for the synthesis of chemicals or pharmaceuticals.
“Think of it as recycling,” said Noémie Elgrishi, assistant professor in the LSU chemistry department. “As fuels are burned or trash is incinerated, they liberate CO2. That CO2, once captured, can be used to re-make fuels or other chemicals, using renewable energy to drive the reactions.”
Something else Louisiana currently has a lot of, other than CO2, is oil and gas infrastructure and expertise. LSU associate professor of petroleum engineering Mehdi Zeidouni sits on the Science Advisory Committee of the Governor’s Climate Initiatives Task Force and is currently involved in two separate projects with industry, bound by non-disclosure agreements, to help leverage the strengths of oil and gas for carbon capture and safe, long-term storage in depleted oil and gas wells and other geological formations, deep underground.
“For oil and gas industry in Louisiana to help cut CO2 emissions, the business model is here,” Zeidouni said. “The industry already has an extensive infrastructure and workforce that can be readily leveraged for carbon capture and storage. Also, given the long history of oil and gas exploration and production in Louisiana, our state has one of the most studied and well-characterized geologic environments in the world. This allows us to minimize the risk of ‘geological surprises’ down in the subsurface where we can’t see. For companies to invest in storage, they need to be able to predict and bank on the outcome, even hundreds of years from now. Oil and gas reservoirs have the capacity to safely store significant amounts of CO2 for the same reason they preserved significant volumes of hydrocarbons for millions of years.”
Zeidouni is also helping industry explore saline aquifers deep underground as potential storage locations, including in Bayou Sorrel and Paradis, Louisiana.
“Where we get the CO2 from is obvious, but where do we put it, and how do we get it there?” Zeidouni asked. “Those are the questions we’re helping to answer. And as far as transportation of CO2 in Louisiana, well, we already have pipelines everywhere—we know how to build a pipeline to transport CO2 throughout the state.”
The Oil and Gas Climate Initiative (OGCI), a global effort in support of the Paris Agreement, has identified the area of Louisiana and Texas as a “high-potential hub,” and possibly the largest, for innovative carbon capture, use, and storage technologies. The Louisiana Mid-Continent Oil and Gas Association (LMOGA) is on board:
“Louisiana is uniquely blessed to have a highly trained, highly skilled workforce that is well suited for building additional pipelines and operating wells for carbon capture and sequestration," said Nathan McBride, regulatory affairs manager at LMOGA. “The state also has highly concentrated industrial corridors that contain pure carbon sources located relatively close to existing CO2 pipeline infrastructure, and ideal geology for storage.”
“LMOGA firmly believes that Louisiana can become a global leader in climate solutions, which LSU is helping to develop, and we welcome the opportunity to prove to the world that energy production and environmental stewardship go hand in hand as investment in this space ramps up,” McBride said.
Note: Yet other LSU scientists, including Kalliat Valsaraj, a professor in the same department as Flake, are working on understanding the fundamental effects of carbon emissions on the atmosphere and near-term climate change, while Keith Hall, professor and director of the LSU John P. Laborde Energy Law Center, sits on a separate task force created by the Louisiana Department of Natural Resources to work on issues relating to carbon capture and storage, aimed at limiting climate change—the LSU Journal of Energy Law & Resources will be hosting a symposium on carbon capture and storage.
Enhanced Oil Recovery
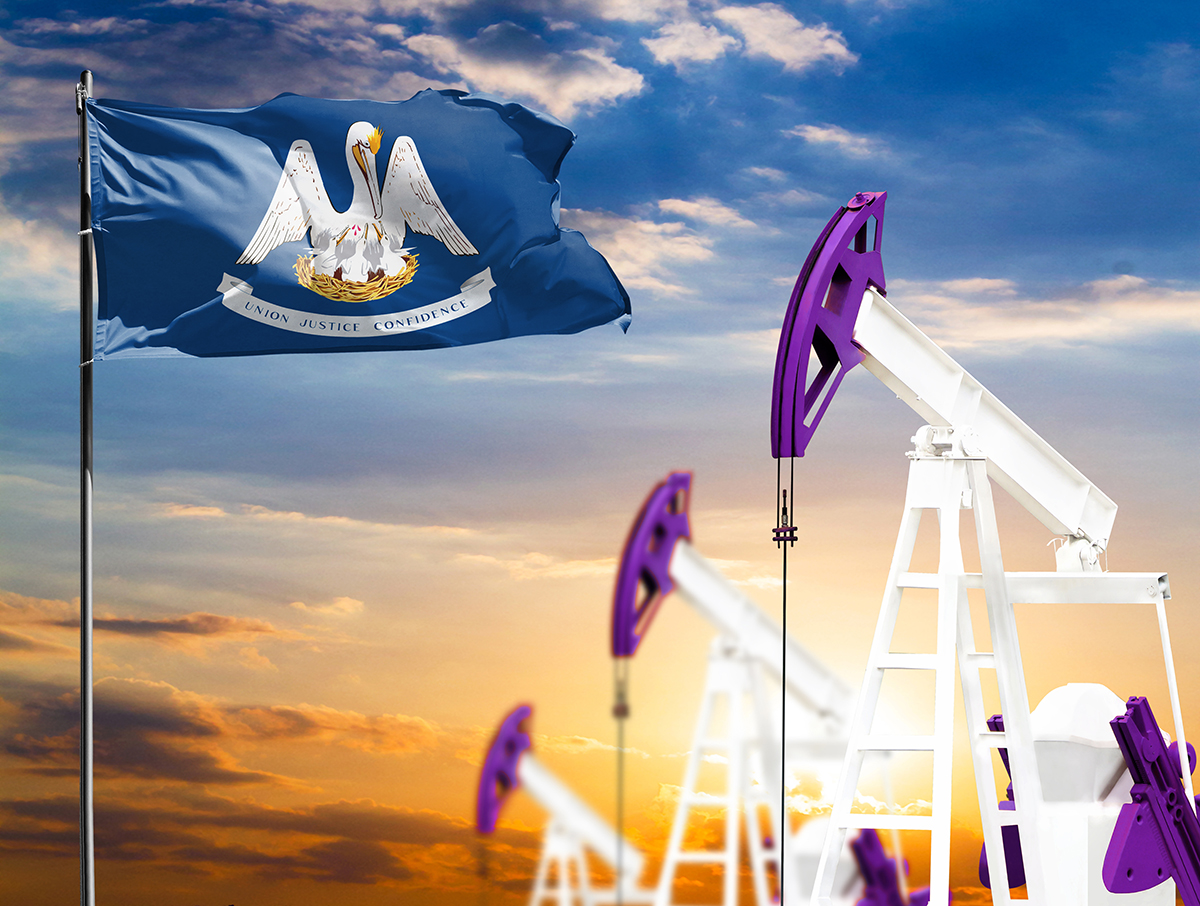
By using CO2 as a tool to harvest more oil from tight shale (while safely and effectively storing it underground), LSU petroleum engineer Dandina Rao sees “tremendous opportunities” in Louisiana.
Oil production has gone down in Louisiana since the 1970s, taking important tax revenues for the state with it. Meanwhile, Texas and other states have experienced an oil boom in recent years, positioning the U.S. as the number-one oil producer in the world. Primarily, this is due to technological advances in oil extraction from tight shale, which now represents 60% of all U.S. oil production.
Dandina Rao, Emmett C. Wells Jr. Distinguished Professor in the LSU Craft & Hawkins Department of Petroleum Engineering, wants Louisiana to benefit from increased recovery from shale, too. A good third of the state—millions of acres all the way from Alabama to Texas—sits upon the Tuscaloosa Marine Shale, which is estimated to hold around 7 billion barrels of untapped oil, “a huge resource.” This is in addition to nearly 13 billion barrels of conventional light oil trapped under our feet in Louisiana, waiting to be harvested using new and improved technologies.
There are challenges and costs associated with getting shale oil out of the ground. Extraction from shale relies on a set of techniques known as “enhanced oil recovery,” where CO2 is injected into the ground at fairly high pressures. The most common, the Water-Alternating-Gas, or WAG, process leads to recoveries around 5-15%, according to Rao. That’s not much.
“With the current technology used in industry today, 90% of the oil is left behind in the rock,” Rao said. “So, we have a tremendous opportunity here...”
—Dandina Rao
“With the current technology used in industry today, 90% of the oil is left behind in the rock,” Rao said. “So, we have a tremendous opportunity here, but instead of trying to improve a process that doesn’t work that well, we decided to think out of the box and came up with an entirely different process.”
Rao and LSU hold two U.S. patents (2012, 2020) for Gas-Assisted Gravity Drainage, or GAGD, which Rao developed at the university with support from the U.S. Department of Energy and the Louisiana Board of Regents. In various lab experiments and recent field simulations in Louisiana and Iraq, this patented process has led to recoveries around 65-99%. A successful field test was also recently done in Vietnam.
An important difference between WAG and GAGD is that the latter doesn’t require pure CO2 for injection, since the gas doesn’t have to mix with the oil under very high pressures (making it “miscible”) for extraction. To get pure CO2 from industrial emissions (refineries, chemical plants, power plants, fertilizer plants, etc.), the gas must first be separated from nitrogen, a natural component of flue gas (what comes straight out of the chimney). This separation accounts for about 75% of the cost of WAG. With GAGD, meanwhile, flue gas can be injected directly, as-is, and then remain in the reservoir, making this enhanced oil recovery process a profitable type of carbon capture, use, and storage. The recently increased 45Q tax credit is helping as well, drawing additional interest in enhanced oil recovery.
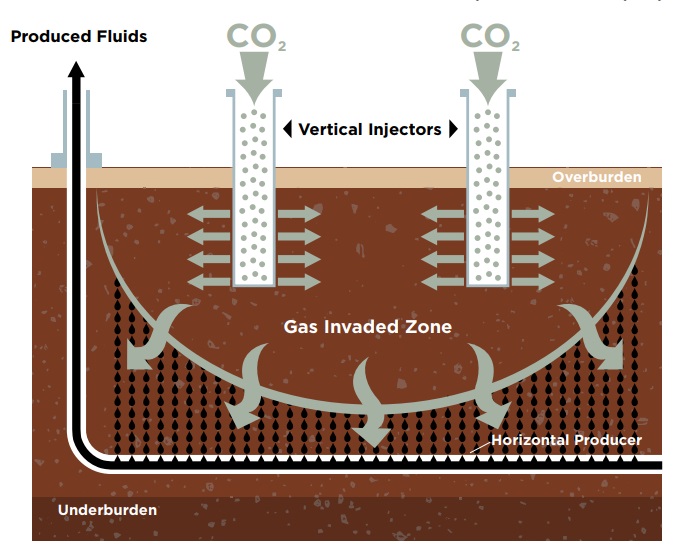
In Rao’s and LSU’s patented enhanced oil recovery process, called GAGD (short for gas-assisted gravity drainage), pressurized carbon dioxide or flue gas is injected underground, pushing oil and water into horizontal producers. While the method most often used in industry today (WAG, or water-alternating-gas) recovers only 5-15% of the oil in tight shale, Rao has observed recoveries around 65-99% in recent field simulations using GAGD.
– LSU
“Using our method, we save the cost of separation of the gases, we get a lot more oil, and we get it out continuously because we don’t have to stop to inject water every so often,” Rao explained. “Also, we can do this at reasonable pressures and, importantly, we don’t fight with nature, creating layers of gas and water and oil. With GAGD, we just inject the gas, which then rises in the shale, allowing oil and water to trickle down and basically rain into our horizontally drilled producers.”
Rao holds another patent for an innovative technology, called Hydroflame, where natural gas is burned inside a cylinder of rotating water, creating both flue gas for injection as part of the GAGD process, and steam. The heat energy in the steam can be converted to pressure energy to run the compressors used for gas injection, or used for power generation for immediate use or storage.
Rao would be driving down the Mississippi River at night and watch the flares from the oil refineries making rural Louisiana look like Manhattan, New York. Louisiana light crude naturally separates into petroleum and natural gas—gas is part of the oil but routinely burned or flared at the plant since there often is no infrastructure in place to store and transport significant amounts of natural gas on the petroleum side, and methane, the primary component of natural gas, is about 25 times more harmful for the environment than CO2 and therefore cannot be released into the atmosphere directly. In 2019, 293 billion cubic feet of natural gas was flared from the Permian Basin alone, the largest source of shale oil in Texas.
“What a waste; it’s a terrible waste,” Rao remarked. “But using our technologies, they could use Hydroflame to do something with the gas, capture the heat energy, and generate flue gas for injection to get more oil out.”
“The world has looked down upon petroleum engineering because they think we are the polluters of the atmosphere and the destroyers of the environment,” Rao continued. “But oil and gas companies have actually shown a lot of interest in various methods of carbon capture, and as a society we’ll continue to need fossil fuels for chemical manufacturing. With our new technologies, we can have it both ways—economic benefit and environmental cleanliness.”
Dandina Rao was elected a senior member of the National Academy of Inventors in 2019, an acknowledgement of his life-long work to find profitable, environmentally sound, and sustainable solutions for oil extraction in Louisiana.
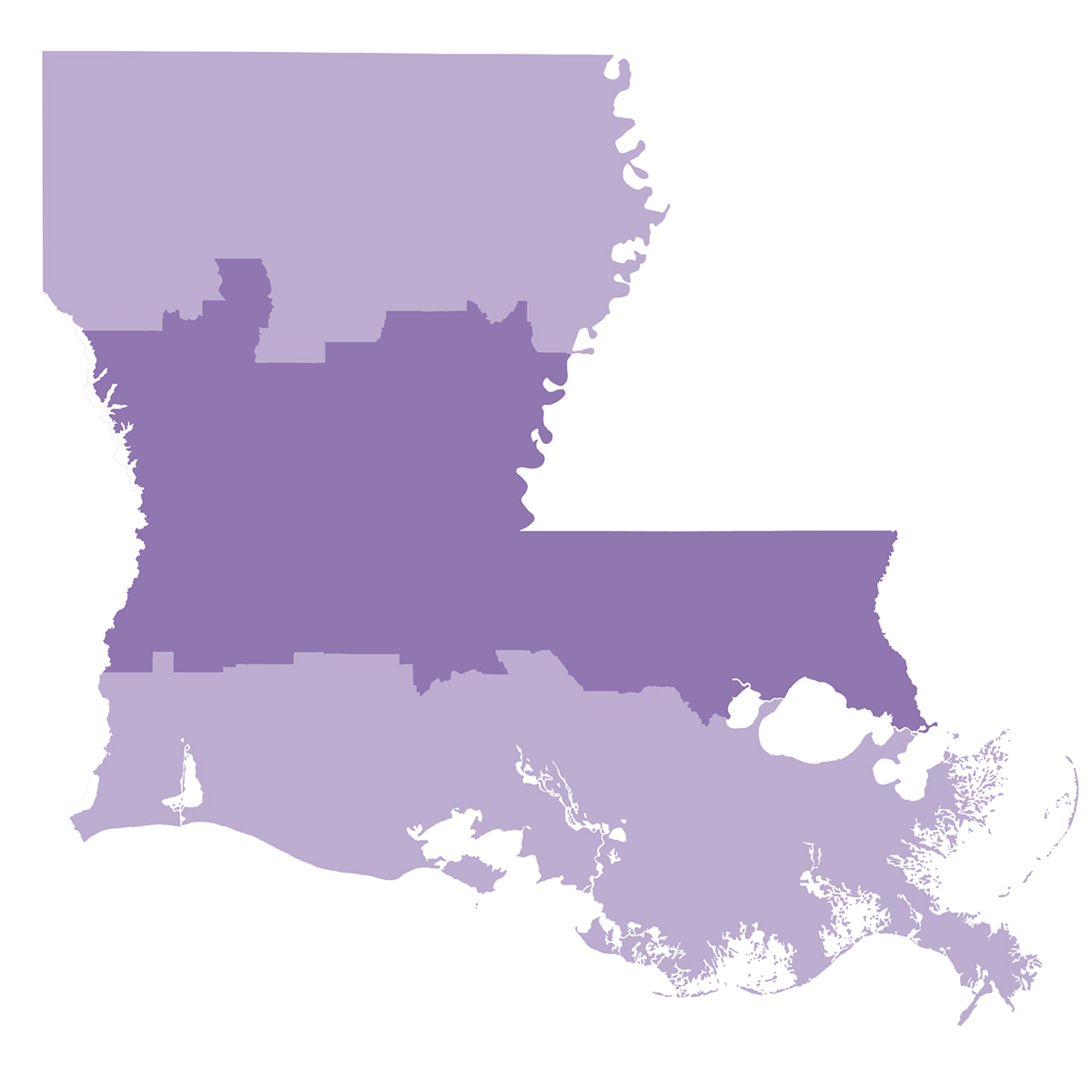
The Tuscaloosa Marine Shale, which stretches right across Louisiana several thousand feet underneath 23 parishes, is estimated to hold around seven billion barrels of untapped oil, “a huge resource.”
“Industrial carbon capture combined with enhanced oil recovery provides a golden opportunity for our state where our petroleum engineers can use their skills and expertise in pipelines, drilling, and reservoirs to get a lot more oil out of the ground and do it in a way that’s good for the environment.”—Ray Lasseigne, TMR Exploration, Bossier City, Louisiana
Someone Rao considers a likeminded friend is Ray Lasseigne, co-owner and president of the oil and gas company TMR Exploration in Bossier City, Louisiana. TMR has an ongoing carbon dioxide-enhanced oil recovery project in Livingston Parish, east of Baton Rouge. There, TMR is closing the carbon loop by separating the CO2 from the produced oil and water and then reinjecting the CO2 right back into the oil reservoir at a depth of 10,400 feet. (The CO2 originally came from the Jackson Dome in Mississippi, the largest naturally occurring CO2 accumulation east of the Mississippi River, specifically for enhanced oil recovery in Livingston Parish.)
“Industrial carbon capture combined with enhanced oil recovery provides a golden opportunity for our state where our petroleum engineers can use their skills and expertise in pipelines, drilling, and reservoirs to get a lot more oil out of the ground and do it in a way that’s good for the environment,” Lasseigne said. “Louisiana has all of the components necessary to do this, and I think LSU is the only entity with the ability to bring all of the pieces together for us.”
Lasseigne has done several projects with senior petroleum engineering students from LSU (both the A&M campus in Baton Rouge and LSU Shreveport offer petroleum engineering programs).
At present, TMR is harvesting about 500 barrels of oil per day in Livingston Parish.
“It’s a closed loop, it’s profitable, and there’s a tremendous need for more of it,” Lasseigne said. “The limit on enhanced oil recovery in Louisiana right now is that there isn’t enough CO2 readily available at a reasonable price, so we have a niche industry waiting to be created to get CO2 from the industrial facilities we already have and putting it into a shared pipeline. We can use the 45Q tax credit to build it, and I think it all starts with LSU—from petroleum engineering to civil and mechanical and chemical engineering to business and law. If we work together, we have all of the pieces. This is fertile ground.”
Blue Carbon
“Plant a tree,” they said. “To help fight global warming, plant a tree.”
Trees and plants consume water and CO2 from the air to grow biomass while releasing oxygen back into the atmosphere. This simple, light-driven process—photosynthesis—supports all life on Earth.
But not so fast, says LSU researcher and former director of Louisiana Sea Grant Robert Twilley:
"All trees are not created equal.”
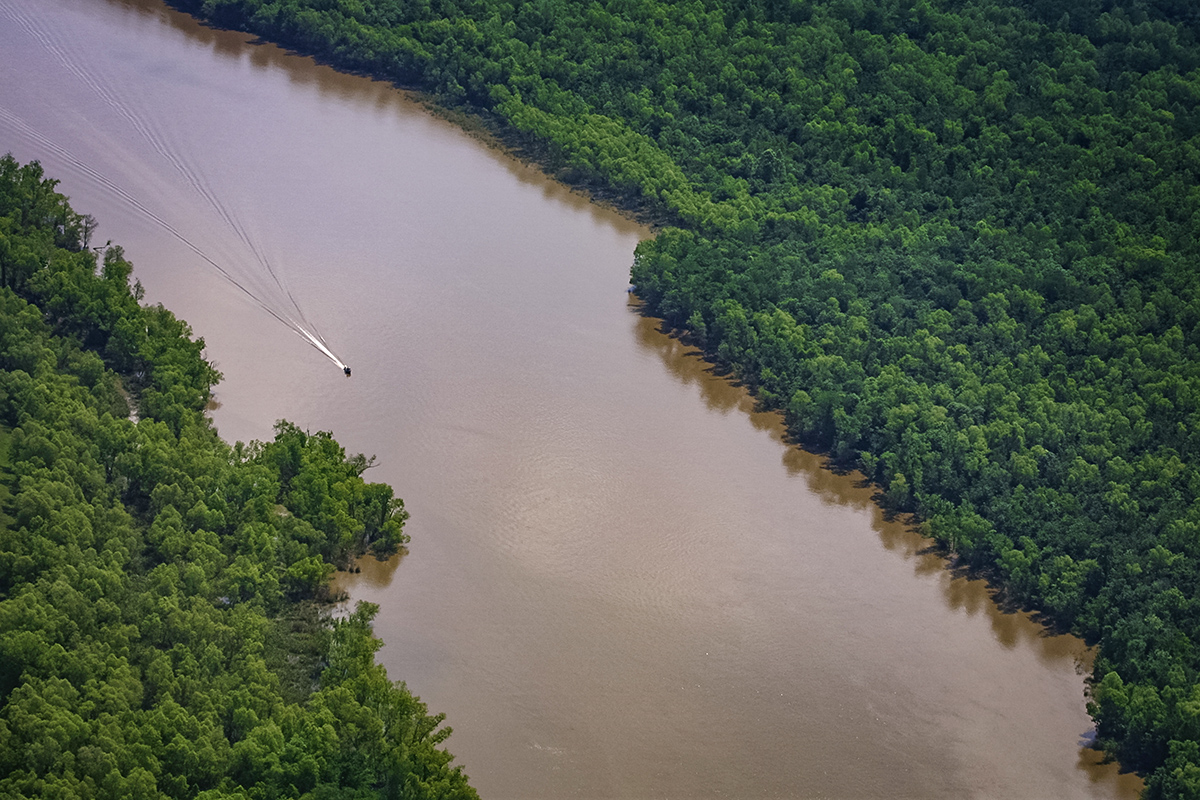
With rising seas, mangroves are expected to thrive in the deltaic areas of Louisiana, such as in the Atchafalaya River Basin.
Although coastal habitats cover only 2% of the total ocean area, they stockpile about 50% of the carbon in ocean sediments.
For over 40 years, Twilley and colleagues at LSU have studied mangroves, a coastal super-tree that thrives in saltwater and deltaic areas around the globe. What makes it “super” is its ability to grab CO2 and store the carbon in its leaves, wood, roots, and—importantly—soil. In deltaic areas where sediment is brought by rivers to the sea, new layers of soil and mud can accumulate quickly, sequestering more carbon. Although coastal habitats cover only 2% of the total ocean area, they stockpile about 50% of the carbon in ocean sediments.
It’s the intersection of land and water that does the trick—the vegetation, the layering of sediment, and an oxygen-depleted (anaerobic) wetland environment where dead plants and trees take a long time to decompose—effectively trapping the carbon instead of releasing it back into the ever-churning carbon cycle where eating, burning, and otherwise consuming organic matter (by humans, animals, bugs, or bacteria) lets CO2 back into the atmosphere.
“You have to look at the net,” Twilley said. “You have the carbon sequestration rate, and then you have the storage. It’s like a savings account. Maybe you have a lot of money in your account, but if you don’t add or spend, the economy doesn’t care. Maybe you’re depositing a lot but spending at the same rate—then it’s just a big flux number. If you want to build wealth, it matters a great deal how much you put into your account on a regular basis—while saving. Mangroves are excellent at this.”
Twilley was part of the global research effort that established the first carbon budget for mangroves in 1992. He’d attended a scientific meeting in Germany about two years prior. Researchers had just calculated how much carbon was being emitted into the atmosphere, how much was up there in the air, and how much was down here on land and in the oceans—and the numbers didn’t add up. The concentration of CO2 in the atmosphere should have been higher than it was. So, where did the carbon go? Twilley harbored a hunch, based on his own measurements.
“‘The Missing Sink,’ was the title of the paper, I think,” Twilley recalled. “So, ecologists from around the world gathered and each got up to present their numbers, and then I got up and the note-taker for the session wouldn’t even type. ‘These numbers don’t matter.’ He ceremoniously closed his 50-pound laptop, and then, as I sat down, he opened it back up again to take notes from the other groups. It was a trip.”
By adding up the carbon numbers from each of the tropical wetland areas, Twilley blew everyone away. Wasn’t this the bigger number they were looking for?
“... working with nature provides us with the least expensive forms of carbon dioxide removal.”—Victor Rivera-Monroy
In the 30 years since, Twilley and colleagues in the LSU College of the Coast & Environment and the LSU School of Renewable Natural Resources have been studying how much “blue carbon” (blue because ocean-based/coastal) is being stored along the coast of Louisiana and in wetlands around the world, and how we can use natural systems, through geoengineering, to help fight global warming. And it’s not just mangroves where land meets ocean in Louisiana; it’s also the cypress-tupelo wetland forests at the marsh-forest boundary.
“You cannot manage a resource if you don’t know how much you have,” said Victor Rivera-Monroy, associate professor in the LSU Department of Oceanography & Coastal Sciences and a former student of Twilley’s. “Quantifying is important because otherwise we don’t know what we’ll lose if we don’t protect our wetlands. For example, we’ve lost about a third of all mangroves around the world in the past 50 years.”
“And at what cost—that’s really the question,” Rivera-Monroy continued. “We know there is a value, but there is no price. If we valued carbon more accurately in the present socio-economic conditions, we could set a price, and that’s important for policy and establishing a carbon market so we know, ‘This is what it costs to emit CO2,’ ‘This is what it costs to destroy mangroves,’ and ‘This is what it costs to fix climate change.’ As systems ecologists, you could say we’re flying a plane and we’re still building it as we go along. It’s kind of dangerous, but at the same time, it is helpful. And working with nature provides us with the least expensive forms of carbon dioxide removal.”
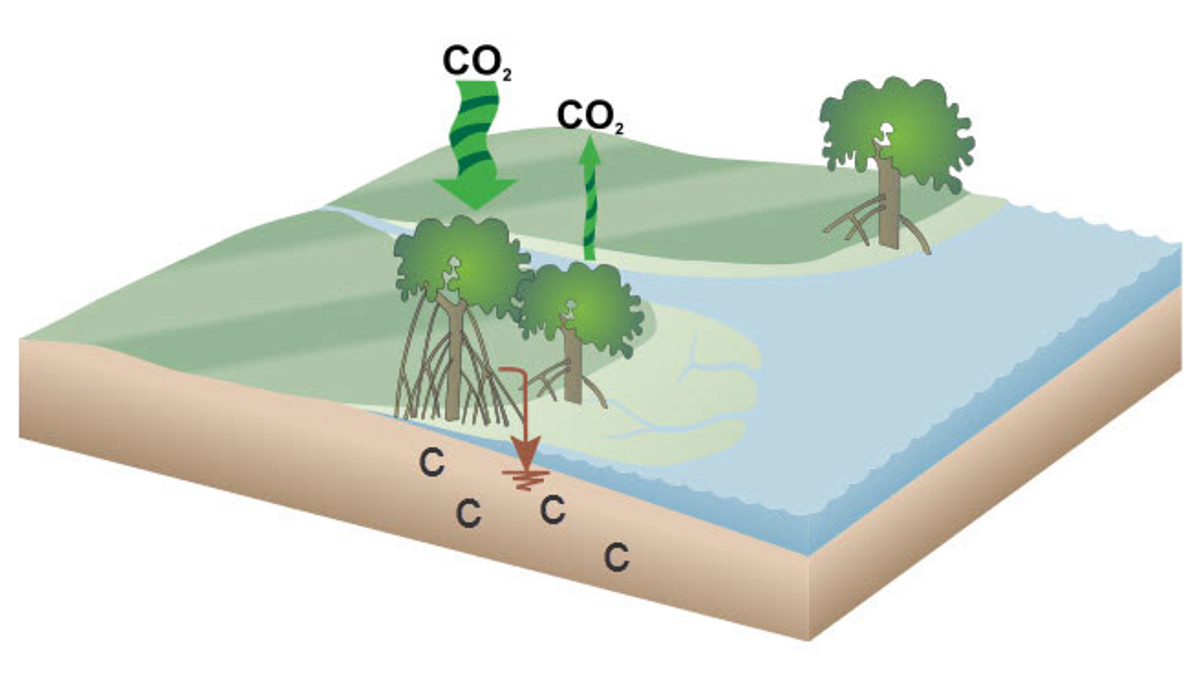
Mangroves are coastal super-trees that thrive in saltwater and deltaic areas around
the globe. What makes it “super” is its ability to grab CO2 and store the carbon in its leaves, wood, roots, and—importantly—soil. Through geoengineering
and by working with and protecting our wetlands and mangrove habitats, Louisiana can
help lead the fight against climate change.
NOAA
LSU researchers are working with industry, including oil and gas companies, and state and local government to protect Louisiana’s wetlands, which not only store carbon, but protect coastal communities against storm surge, and support key fisheries.
“Oil and gas companies have a vested interest in protecting our wetlands and investing in blue carbon projects because they have to protect their facilities,” Rivera-Monroy said. “They have billions of dollars in infrastructure that is threatened by sea level rise, due to climate change. They want to transfer into green energy and have plans for that, but the transition is going to take 10 to 30 years. So, that’s a major issue, yet during this transition, we can also use wetlands to capture CO2 by working with natural processes regulated by currents and sediment transport that help maintain marshes and other forested wetlands, including mangroves.”
The Mississippi River can be used as an engine to drive sediment to increase carbon sequestration. It also brings nutrients, which allow mangroves to grow bigger and faster and store more carbon in their wood, which sets them apart from most aquatic plants.
“Mangroves in deltaic coasts such as the Mississippi River Delta can sequester more carbon yearly than any other ecosystem on the globe,” Twilley said. “These are the world’s blue carbon hot spots.”
Instead of basing a global carbon price on guesstimates of damages, [Brian] Snyder suggests, why not base it on the cost of implementing solutions?
Interestingly, climate change—higher temperatures and rising seas—will likely lead to more mangroves in Louisiana, like in the Florida Everglades. There’s other potentially good news, too. Rising seas could help mangroves sequester more carbon, as long as sediment is available to maintain their elevation. Similarly, in a recent study Rivera-Monroy did on submerged aquatic vegetation with his LSU colleagues Megan La Peyre, Eva Hillman, and Andrew Nyman, the researchers found a previously unaccounted-for pool of carbon in the muddy waters of Louisiana, home to 40% of the nation’s coastal wetlands.
“You look into the clear waters in Florida and you see the seagrasses, and we know how important seagrasses are for carbon sequestration, but I think a lot of people in Louisiana just assumed—because it's muddy here and plants need light to do photosynthesis—that it’s not the same,” Rivera-Monroy said. “But there are plants that have adapted to be able to capture the light in muddy-but-shallow areas, and that’s approximately 108 square miles in southeast Louisiana across the Mississippi River Delta Plain, including key areas, such as Pontchartrain, Barataria, and Terrebonne watersheds.”
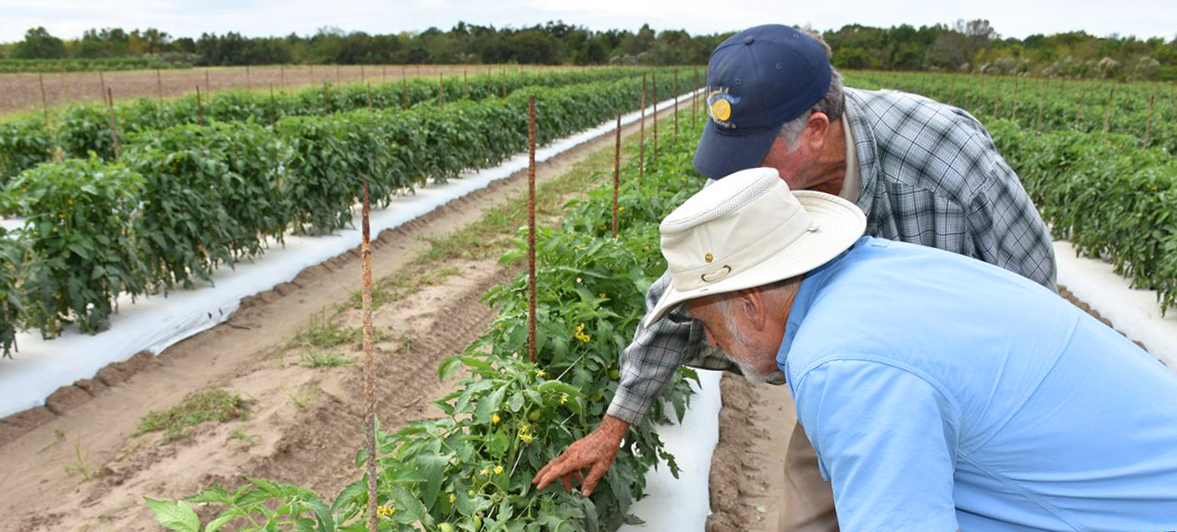
Leveraging Louisiana’s Natural Resources:
Working with Our Farmers
One-fourth of all greenhouse gas emissions come from land-use practices, such as farming. As an agriculturally dominant state, Louisiana can play a critical role in CO2 mitigation through appropriate land management practices that enhance carbon sequestration in soil, which is something the LSU AgCenter’s research faculty and extension agents are working on together with thousands of Louisiana farmers.
By collaborating with the Louisiana Department of Agriculture and Forestry, Natural Resources Conservation Service, and the Environmental Protection Agency, the LSU AgCenter is helping to establish agricultural best management practices, offering carbon-conscious guidelines on cover crops, reduced tillage, crop rotation, and grazing management, which also contribute to higher productivity and profitability.
The team measured short-term carbon storage in the plants themselves and long-term carbon storage in the soils where they grow—almost as much carbon as in mangrove soils, seagrasses, and salt marshes, well ahead of tropical and temperate forests.
“This might help offset the loss in carbon sequestration that comes with marsh and wetland loss due to erosion, subsidence, river diversions, and relative sea level rise—all of the new, shallow open-water areas are potential habitats for submerged aquatic vegetation,” remarked Rivera-Monroy.
He has frequent conversations with his colleague down the hall, Brian Snyder, about ways to allocate monetary value to natural systems, so they can be regulated and managed in ways that might make sense for future generations.
As a warming world tries to come to grips with how to establish a carbon market—potentially leading to a global cap and trade system with fair compensation for those who invest in solutions to lower the CO2-concentration in the air as well as those who will suffer the most immediate and long-term effects of climate change—the term “social cost of carbon” (SCC) is often used. In a recent paper, Snyder raised several issues with this concept, however. First, it’s an attempt to (ambitiously) account for all damages to human systems. As such, impacts without price tags are easily ignored. Climate change is predicted to lead to malnutrition, migration, and increased conflict—especially in third-world countries whose economies are entirely separate from those responsible for the largest shares of CO2-emissions, such as the U.S. and China—how do we accurately “charge” or compensate for that? Human systems also do not equate to the whole biosphere (an if-a-tree-falls-in-the-forest problem, especially if the forest isn’t owned by anyone). Instead of basing a global carbon price on guesstimates of damages, Snyder suggests, why not base it on the cost of implementing solutions? Now that we have technologies to remove CO2 from the atmosphere, so-called NETs, or negative emission technologies, the price of carbon could be linked to clear and verifiable costs.
“We’re already familiar with the Polluter Pays Principle, so this approach should make more sense to people,” Snyder said. “If I spill a bunch of oil into the Gulf of Mexico, I'm required to clean that up, right? But at present, if I release a bunch of carbon dioxide into the atmosphere, there’s no requirement for me to clean it up, and there’s also no payment that I have to make to the government or somebody else to clean it up in the future.”
“Without a price on carbon, ecosystem services such as carbon sequestration in wetlands and mangroves are always going to be undervalued, and that’s the issue,” Rivera-Monroy added. “With a polluter-pays system, we could also recognize that there is a cultural and even spiritual value in the preservation of our environment that we simply cannot put a price on and trade on the stock market.”